Would More Electric Vehicles Be Good for the Environment?
Executive Summary
The environmental advantages of electric vehicles (EVs) over internal combustion engine-powered vehicles are as obvious as the lack of a tailpipe emitting air pollutants and carbon dioxide, but there are environmental disadvantages as well. These disadvantages would become far more serious should policy makers choose to take EVs beyond their current niche status and make them a major component of America’s 17 million per year new vehicle market.
Producing a battery for an EV requires many mined materials, including lithium, cobalt, and rare earths. Most of this is mined and processed in nations like China, Congo, and Chile, where environmental standards are weaker than in the U.S. While America has deposits of many of the required materials, domestic mining is made difficult by environmentalist opposition, including by organizations that simultaneously advocate for more EVs.
Beyond the local impacts of mining and processing on land, air, and water, the energy that goes into making an EV battery is more than that needed for a conventional vehicle engine and results in greater carbon dioxide emissions during the manufacturing stage. This so-called carbon debt is incurred by each EV before it is even driven its first mile and may take years to repay.
Replacing gasoline with electricity as the energy source for personal transportation does not eliminate emissions of air pollutants and carbon dioxide so much as displace them. If coal-fired electricity were to continue to be a significant part of the generation mix, then the emissions reductions from the transition may be minor and possibly nonexistent. But even if a transformation of the vehicle fleet to EVs is accompanied by an equally difficult buildout of renewable electricity generation, there will still be significant environmental impacts.
Producing many more EVs will require dealing with many more spent EV batteries, which pose a number of environmental challenges beyond those associated with the lead-acid batteries in conventional vehicles. Recycling an EV battery is far from simple and poses a number of environmental tradeoffs.
Policy makers should consider these and other environmental costs before they take any steps towards locking the nation into a future of electric vehicles as a major means of transportation.
Introduction
Electric vehicles (EVs) have been a favorite of environmentally minded policy makers for decades. They have certain obvious environmental advantages over internal combustion engine (ICE) vehicles—no reliance on petroleum-based fuels and no tailpipe emissions of air pollutants or carbon dioxide. EVs have an environmental downside as well, but it is much less obvious and therefore has been largely ignored to date.
Consumer acceptance of EVs is hampered by two major disadvantages compared to ICE vehicles—higher costs and limited range before an hours-long recharge is needed. While sticker prices are coming down and range is improving, progress has been slow. On the other hand, EVs benefit from generous federal and state tax credits and other incentives, and sales have increased in percentage terms.1
However, the nearly 2 million EVs on American roads today—roughly half in California—are still a very small percentage of the total of over 200 million cars and trucks in use. Their annual sales in the 300,000 to 400,000 range are only a small percentage of the total of 17 million new vehicles— predominantly SUVs and pickup trucks—sold in recent years.2
Niche status for EVs is not good enough for those who consider climate change an existential threat, especially since transportation contributes nearly one-third of American emissions of carbon dioxide.3 Many are now calling for much more aggressive measures, including mandates, to eventually make EVs the predominant—or perhaps only—choice in new car sales. The first such measure to reach a vote in Congress was the 2019 Green New Deal resolution.4 While the bill’s text was somewhat ambiguous as to its greenhouse gas reduction requirements for new vehicles, an explanatory document released along with the resolution called for a “goal to replace every combustion-engine vehicle” over a 10-year span.5
Table 1. Electric Vehicle Sales Compared to Total Vehicle Sales, 2018 and 2019
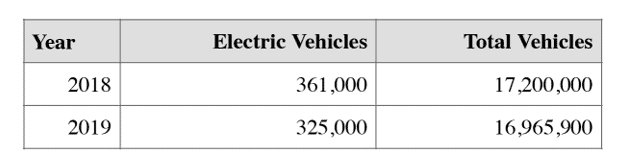
Source: Department of Energy, Office of Energy Efficiency and Renewable Energy, Vehicle Technologies Office, https://www.energy.gov/eere/vehicles/articles/fotw-1124-march-9-2020-us-all-electric-vehicle-sales-level-2019. Department of Energy, Office of Energy Efficiency and Renewable Energy, Vehicle Technologies Office, https://www.energy.gov/eere/vehicles/articles/fotw-1116-january-13-2020-us-light-duty-vehicle-sales-2019-were-nearly-17. Department of Energy, Office of Energy Efficiency and Renewable Energy, Vehicle Technologies Office,
The Green New Deal proved unpopular, and the Senate vote on it failed spectacularly—even its cosponsors voted present rather than yes. The House version was never brought to a vote. However, it is not unusual for major environmental bills to fail on the first try, and such measures can be expected to come up again.
Supporters of expanded EV sales can look to those already in use and say “so far, so good.” The compounds needed to make them have been readily available on the global market, the modest additional electricity demand has been met by the existing grid, their relative few owners are satisfied with the fueling infrastructure available to them, and the disposal of old batteries has not yet caused major issues. But all this would change dramatically were EV production and sales to be scaled up by a factor of 10 or more, which would be necessary for them to meet their proponents’ greenhouse gas reduction goals. In other words, the hidden environmental downside could become too large to hide.
This analysis focuses on the effects of expanded production of electric vehicles, assuming that other putative “zero emission” technologies, such as hydrogen fuel cells, do not capture significant market share and that federal regulators will increasingly treat hybrids as internal combustion engine vehicles and not encourage their adoption as much as that of fully electric vehicles.
Increased Global Mining and Attendant Environmental Impacts
For the most part, manufacturing an electric vehicle is similar to manufacturing an internal combustion engine vehicle, the major exception being the powertrain. In many respects, producing a battery for an EV is more energy- and resource-intensive than making a comparable conventional vehicle engine. The potential cumulative environmental impacts of producing millions of such batteries annually has received scant attention thus far.
A state-of-the-art lithium-ion EV battery weighs about 1,000 pounds. This includes about 25 pounds of lithium, 30 pounds of cobalt, 90 pounds of copper, 110 pounds of graphite,
60 pounds of nickel, and the rare earths neodymium and dysprosium.6 Most of this is mined and processed outside the U.S., and much under conditions that raise serious environmental and safety concerns. According to forecasts from the financial firm UBS, replacing global sales of ICE vehicles with EVs would require a projected 2,898 percent increase in lithium, a 1,928 percent increase in cobalt, a 524 percent increase in graphite, a 105 percent increase in nickel, and a 655 percent increase in rare earths.
Table 2. Percentage Increase in Mined Materials for an All-Electric Vehicle World
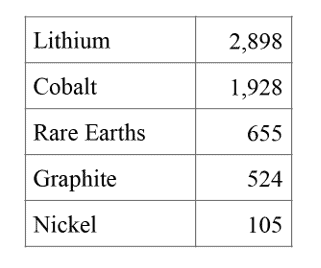
Source: Jamie Smyth, “BHP positions itself at centre of electric-car battery market,” Financial Times, August 9, 2017, (subscription required). https://www.ft.com/content/367149e8-7ca2-11e7-ab01-a13271d1ee9c
Current mining and processing to produce today’s relatively small EV volumes is environmentally problematic enough and creates a worrisome baseline. Lithium is mined relatively safely in Australia, but the largest reserves are in South America, led by Chile, where it exists in brine rather than ore. Among other things, extraction and purification require a lot of water in the most arid part of the South American continent.7 Beyond water shortages that have forced out farmers and ranchers, lithium mining in South America causes “ecosystem degradation” and “landscape damage,” according to a recent United Nations report.8
Congo dominates the world market for cobalt, and its mining and processing is the stuff of environmentalist and human rights group crusades.9 Along with serious safety and child labor issues, the U.N. report notes acid mine drainage that “pollutes rivers and drinking water,” as well as dangerous air emissions.10
China figures prominently in the mining and processing of most materials required to make EV batteries. This includes domestic activity and substantial investments in mines and processing facilities around the globe. China has a commanding share of the global market for most of the necessary inputs, particularly for lithium, cobalt, graphite, and rare earths.11 As with much Chinese- directed industrial activity, most of this mining and processing is subject to minimal environmental protections and is largely powered by coal.
These elements are typically found in very low concentrations—in many instances only a fraction of 1 percent in the ores mined—so each EV battery requires the mining and processing of literally tons of earth above and beyond that of an ICE vehicle. By one estimate, 250 tons of ore are needed for each battery.12
It is important to note that the expansion of mining to meet sharply increased demand for EV batteries would likely occur in a non-linear fashion. For the most part, the most productive deposits are the ones currently being exploited. New mining will likely require more land per unit of output, as well as more intensive and environmentally damaging processing of lower-grade ores.13 Moreover, many near-surface deposits have already been exhausted, so opening new mines would require the removal of vast amounts of overburden—the material above the resource to be extracted—a process that increases the scale of alterations to the land. Furthermore, as will be discussed in the next section, a scaling up of EVs may occur over the same time frame as an equally ambitious buildout of stationary battery capacity for electricity storage from renewable energy sources, and thus place further pressure on the supply of needed elements.
Technological advances likely will lead to the more efficient use of these materials, especially if they are in response to high prices. Even so, the massive increases that would necessarily occur if sales of EVs were to predominate in the new vehicles market would swamp any such improvements.
A Carbon Debt and the Renewable Fuel Standard Example
Beyond the adverse impacts on the land and water, mining and processing and refining these elements is very energy-intensive. In countries that produce these materials, it usually requires fossil fuels, primarily coal.
This is a part of the reason why electric vehicles emit more carbon dioxide during their manufacture than internal combustion vehicles. Thus, a pro-EV policy creates a counterproductive “carbon debt” before the vehicle is driven its first mile. That debt is eventually repaid by using— presumably—lower carbon electricity rather than gasoline as a fuel source, but even some EV proponents concede that could take up to two years.14 Others believe the break-even point occurs significantly later in an EV’s life.15
A similar carbon debt argument has led some scientists and environmental organizations to question the greenhouse gas reduction benefits of the Renewable Fuel Standard. This federal program, which requires the inclusion of corn-based ethanol and other agricultural products in the liquid fuel supply, is supposed to achieve certain targets for reducing carbon dioxide emissions.16 However, the resultant conversion of carbon-storing grasslands and forested areas into cropland to grow the additional corn needed to produce ethanol releases a great deal of carbon at the outset.
This creates a massive carbon debt that may undercut, or even negate, any subsequent benefit from replacing part of the gasoline supply with ethanol.17 It would have been better had such environmental concerns been fully considered before the Renewable Fuel Standard mandate was signed into law. The same lesson should apply to EV policy.
Domestic Mining off the Table
There is a way to reduce the environmental damage associated with the mining and processing of EV battery components in other countries— conducting more such activity in the U.S., where stricter environmental requirements apply. Furthermore, if we are to see a massive scaling up of EVs, additional U.S. production may become necessary to ensure adequate and reliable supplies and avoid prohibitively high costs.
The potential good news is that America has known deposits of nearly all the materials needed to make EV batteries. However, most are not currently being utilized in part because anti-mining activists—often the same advocacy groups and individuals and groups lobbying for more EVs—have succeeded in making American mining and processing of needed compounds all but impossible.
Vast areas in the West, including geologically promising ones, are federally controlled, and many of them have been placed off limits to mining.18 In addition, federal and non-federal lands where mining can occur face a gauntlet of environmental restrictions. Even those mine projects that successfully navigate the lengthy approval process may come online too late to contribute to any EV buildout within the next decade.
The environmental restrictions on American smelters are no less daunting. Even if more of the needed elements for EV batteries were to be mined in the U.S., the ores may have to be sent overseas for processing.19
Nor is there any evidence of a change of heart over domestic mining in order to facilitate expanded EV production. For example, one recent report from several environmental organizations focuses on domestic deposits of lithium, cobalt, and rare earths, and concludes that most of them are in areas too pristine and ecologically sensitive to disturb.20
Two ongoing events further demonstrate the continued opposition to American mining by EV proponents—the environmental review of Alaska’s Pebble Mine and the Trump administration’s reform of the National Environmental Policy Act (NEPA).
Alaska’s Pebble Mine is near the end of its lengthy federal approval process under NEPA. It has the potential to be America’s most productive new mine in decades, containing vast reserves of many minerals, including several used in EV batteries. Perhaps most significantly, it could prove to be a significant source of the needed rare earths, and thus help keep future prices in check, while undercutting China’s dominance of the global market for them.
The Army Corps of Engineers recently finalized its exhaustive Environmental Impact Statement required under NEPA.21 It concluded that the proposed mine does not pose a serious environmental risk. Nonetheless, virtually the entire environmental activist community opposes the Pebble Mine, and some organizations have already filed lawsuits to block the project and will likely continue to do so.22
Beyond this one mine, NEPA reforms recently finalized by the Trump administration’s Council on Environmental Quality may serve to streamline the approval process for other mines and processing facilities, but are opposed by environmentalist supporters of EVs.23 The environmental reviews of major projects under NEPA average four and a half years, and the final decision is almost always litigated, resulting in further delays.24 For new mines, the permitting process is in the seven- to 10-year range.25 In many cases, the delays are so long that mine developers are forced to give up. For practical purposes NEPA acts as a ban on such projects. Indeed, NEPA is a major reason mining in the U.S. is at a competitive disadvantage compared to countries whose approval process for mines is much less costly and time-consuming.
Among other things, the NEPA reforms would set a deadline of two years on the environmental review process. They also would limit the scope of reviews to reasonably foreseeable environmental impacts of a proposed mine and not highly speculative or tangentially related ones.
Legal challenges to these NEPA reforms have already been filed.26 Whether these reforms withstand judicial scrutiny will go a long way toward determining whether the environmental impacts related to producing EVs can be reduced with more domestic production of battery components.
Large-Scale EV Use
Americans used 142 billion gallons of gasoline in 2019 (about 1,000 gallons per household), most of which was produced at the nation’s 135 refineries.27 This fuel was provided to end users at more than 150,000 retail gas stations spread out along the nation’s roads and highways.28 At any one of those stations, an internal combustion vehicle driver can, in about five minutes, get enough fuel to go another 400 or more miles.
This extensive infrastructure delivers a vast amount of energy, and it does so with a level of convenience and reliability to which Americans have become accustomed and against which alternative vehicles must compete.
Creating something comparable for EVs will impose environmental impacts as well as costs.
Impacts from Electricity Needed to Run EVs
While producing and using 142 billion gallons of gasoline certainly has environmental impacts, so would replacing a significant amount of that energy with electricity. The emissions of air pollutants—chiefly nitrogen oxides, sulfur oxides, and particulate matter—and carbon dioxide are not being eliminated so much as displaced if the electricity used to run EVs includes a significant contribution from coal-fired generation. One study, based on the U.S. Energy Information Administration’s (EIA) 2018 projections of the future electricity mix to 2050, finds that a large-scale switch from ICE vehicles to EVs would lead to slightly higher emissions overall of air pollutants and a less than 1 percent drop in carbon dioxide emissions.29 Others reach broadly similar conclusions.30 Granted, the process of attributing power plant emissions to EVs is far from straight- forward and driven by assumptions, but these studies give reason to question the magnitude of any emissions reductions.
Proponents counter that the scaling up of EVs will be accompanied by an equally ambitious buildout of non-hydroelectric renewable electricity. However, given the many limitations of renewables like wind and solar cost, intermittency, land use, and other factors—relying primarily on them without substantial non-renewable baseload power, or dramatic increases in stationary battery capacity at a cost that could reach into the trillions of dollars,31 is unrealistic and helps explain EIA’s more modest projections of changes to the electricity mix.32
All-Renewable Electricity Would Not Eliminate Environmental Impacts
Even assuming an all-renewable electricity future, there would still be serious environmental impacts. By one estimate, an additional 635 Terawatt-hours or 13 percent more electricity generation would be needed for an all-electric vehicle fleet by 2050.33 If this additional electricity is generated by wind, it would require new turbines spanning 31,000 square miles of land, an area the size of South Carolina.34 Other studies project even larger increases in electricity generation.35
Beyond generation issues, the need for additional high-voltage transmission lines to bring all this new wind and solar into the grid significantly increases the environmental impact.
By one estimate, an all-renewables grid would require a doubling of the 200,000 miles of high-voltage transmission lines in the U.S.36
Adding solar to the renewables mix does not necessarily reduce the difficulties with the transition. As seen with the August 2020 rolling blackouts in California, reliance on solar without sufficient baseload generation or battery storage is problematic after sundown.37 It is anticipated that most EVs would be charged at home and at night.
As noted, the needed buildout of stationary battery capacity would be substantial. By one estimate, reliance on wind and solar in the U.S. would require approximately 900 gigawatts (GW) of storage capacity, compared to a current global total of only 5.5 GW.38 Stationary batteries require many of the same inputs as EV batteries and thus would exacerbate the mining and other environmental issues discussed previously.
For these reasons, a scaling up of EV use leads to an environmental trade- off—either accept additional emissions from coal- and natural gas-fired generation or accept the impacts of greatly expanded renewables and storage.
Charging infrastructure, though mostly an economic concern, would also impose environmental impacts, especially if done on a scale that makes EVs practical beyond a relative few urban centers where most existing home and public charging stations have been placed. Beyond the charging stations themselves, much of the distribution infrastructure will need to be upgraded should EVs expand beyond just a few per neighborhood. These challenges are even greater if charging times are to be reduced to minutes rather than hours via fast-charging capability.
Advances in EVs add to the charging challenge. Longer ranges require larger batteries and potentially longer charging times. In turn, bringing down charging times adds greatly to each EV’s strain on the system. All of this will compound the task of expanding EV usage by tens of millions above current levels.
Table 3. Carbon Dioxide Emissions Trends, Internal Combustion Vehicles
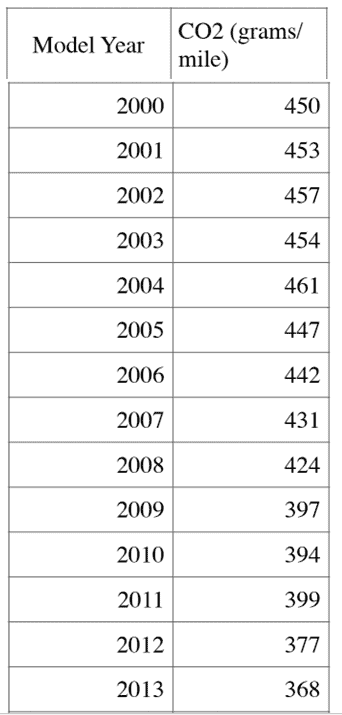
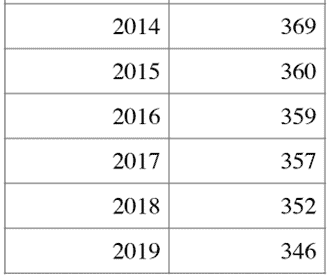
Table 3a. Nitrous Oxide Emissions Standards for Internal Combustion Vehicles
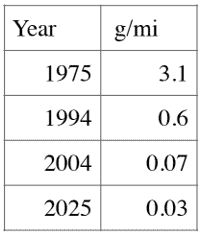
Source: Environmental Protection Agency, Automotive Trends Report,https://www.epa.gov/automotive-trends/download-automotive-trends-report#Report-Tables; Environmental Protection Agency, Light Duty Vehicle Emissions, https://www.epa.gov/greenvehicles/light-duty-vehicle-emissions.
Technological advances will help bring down the emissions profile of each EV, but improvements are also bringing down the emissions profile of ICE vehicles.
In fact, such improvements are required by law. This includes Tier 3 tailpipe emissions standards that replaced the already stringent Tier 2 standards starting in model year 2017, as well as Corporate Average Fuel Economy emission standards set to increase by 1.5 percent annually through model year 2025.39 Thus, it is not clear whether replacing ICE vehicles with EVs will ever deliver reduced emissions. The bulk of the reduction in emissions in the transportation sector over the last two decades has been due to higher fuel efficiency and lower emissions in ICE vehicles rather than the introduction of EVs.40 Any continued declines in ICE vehicle emissions would be jeopardized by measures to push them aside in favor of EVs, as the needed research and development investments would no longer make economic sense.41
Large-Scale Electric Vehicle Disposal
Another challenge facing the widespread adoption of electric vehicles that has not gotten enough attention concerns end-of-life issues. While more than 10 million discarded conventional vehicles—including their lead-acid batteries—are handled each year without serious costs or environmental problems, doing so will not be so easy for EVs. Many of the same environmental risks regarding the chemicals that go into the manufacture of EV batteries also complicate their disposal. And if millions of new EV batteries are to be made annually, then millions of old ones will need to be dealt with annually as well.
EV batteries degrade and eventually become unusable for propelling a vehicle, typically after about eight years of use. Simply disposing of millions of such batteries each year, either immediately after their use in vehicles or after some secondary purpose such as a stationary battery, presents a number of problems. Many of the components pose groundwater contamination risks and thus may not be suitable for conventional landfills. Illegal dumping may also become an issue. There are also safety concerns, as discarded EV batteries may still hold a dangerous charge. One industry representative noted in Senate testimony that without safe disposal, “it is not a question of if a child wandering through a field or junk yard will be electrocuted, but how many and how long before we decide to do something about it.”42
The other option is the recycling of old batteries to provide the materials for new ones. Many optimistically see this as a way to reduce the need for mined materials.43 However, there are challenges to EV battery recycling similar to those already being experienced in dealing with the e-waste from tens of millions of discarded computers and cell phones each year.
It is important to note that recycling is itself an industrial process. The only difference from other processes is that one or more inputs come from a previous use rather than primary production.44 And recycling may not necessarily be the environmentally superior choice.
It is not a simple matter of pulling out and separating the various components from a junked EV battery for reuse in a new battery. Their complex composition does not lend itself to easy disassembly into reusable materials, as is the case with lead acid batteries.45 The lack of standardized EV battery designs further complicates recycling efforts.
There are a number of recycling processes to choose from, each with its own environmental advantages and disadvantages.46 Some use more energy than others, some require more chemical inputs, some create more emissions than others, and some yield more usable recycled material than others. As with much e-waste, making an EV battery from recycled materials is currently not cost-competitive with making one from scratch, so doing so requires subsidies, mandates, or both.48
The question of greenhouse gas emissions is similarly murky.
Notwithstanding the energy-intensive nature of the mining and processing of the materials needed in batteries, it is unclear whether replacing virgin in- puts with recycled ones will reduce emissions or increase them.48
There are also worker safety issues, which helps explain why so much e-waste recycling is offshored to developing nations.49 The same may be the case for EV batteries.
Nonetheless, regardless of where these spent batteries end up, they pose an environmental risk at the end of their useful life that should not be ignored when considering any policy for scaling up EV use.
Conclusion
The environmental advantages of electric vehicles over internal combus- tion vehicles may be as clear as the lack of a tailpipe, but there are disadvantages as well. Manufacturing an EV requires more energy inputs and imposes more mining-related impacts than an ICE vehicle. Using electricity rather than gasoline as a fuel source changes the environmental footprint but does not eliminate it.
And disposing of old EV batteries creates environmental risks beyond those of conventional ICE vehicle batteries. Each of these concerns would intensify with increased production and use of EVs beyond the relative few on American roads today. The environmental downside should be fully thought through before any pro-EV policy is finalized and implemented.
NOTES
1 Jonathan Lesser, “Short Circuit: The High Cost of Electric Vehicle Subsidies,” Manhattan Institute, May 15, 2018, https://www.manhattan-institute.org/html/short-circuit-high-cost-electric-vehicle-subsidies-11241.html.
2 Department of Energy/Office of Energy Efficiency and Renewable Energy, “All-Electric Vehicle Sales Level Off In 2019,” March 10, 2020,
https://www.energy.gov/eere/vehicles/articles/fotw-1124-march-9-2020-us-all-electric-vehicle-sales-level-2019. Statistica, “Light Vehicle Sales in the United States From 1978 to 2019,” 2020,
https://www.statista.com/statistics/199983/us-vehicle-sales-since-1951/.
3 U.S. Environmental Protection Agency, “Sources of Greenhouse Gas Emissions,” 2020, https://www.epa.gov/ghgemissions/sources-greenhouse-gas-emissions.
4 S. Res. 59, A Resolution Recognizing the Duty of the Federal Government to Create a Green New Deal, 116th Congress, First Session, February 7, 2019,
https://www.congress.gov/bill/116th-congress/senate-resolution/59/text?q=%7B%22search%22%3A%5B%22green+new+ deal%22%5D%7D&r=2&s=1.
5 Green New Deal Frequently Asked Questions, February 7, 2019, https://assets.documentcloud.org/documents/5729035/Green-New-Deal-FAQ.pdf.
6 Mark Mills, “Mines, Minerals, and “Green” Energy: A Reality Check,” Manhattan Institute, July 2020, p.7, https://media4.manhattan-institute.org/sites/default/files/mines-minerals-green-energy-reality-checkMM.pdf.
7 Amit Katwala, “The Spiralling Environmental Cost of Our Lithium Battery Addiction,” Wired, August 5, 2018, https://www.wired.co.uk/article/lithium-batteries-environment-impact.
8 United Nations Conference on Trade and Development, “Commodities at a Glance: Special Issue on Strategic Battery Raw Materials,” 2020, https://unctad.org/en/PublicationsLibrary/ditccom2019d5_en.pdf.
9 Amnesty International, “Amnesty Challenges Industry Leaders to Clean up their Batteries,” March 21, 2019, https://www.amnesty.org/en/latest/news/2019/03/amnesty-challenges-industry-leaders-to-clean-up-their-batteries/.
10 Ibid.
11 Matthew Bolsen, “Control the supply chain, control the world—The battle for critical materials and rare earths begins…,”
InvestorIntel, July 10, 2020,
https://investorintel.com/sectors/technology-metals/technology-metals-intel/control-supply-chain-control-world-battle-critical- materials-rare-earths-begins/.
12 Mills, p. 7.
13 Bloomberg, “Elon Musk Has a Big Dream to Power Tesla Cars, but Reality May Be a ‘Rough Cut,’” Hindustan Times, August 22, 2020,
https://auto.hindustantimes.com/auto/news/elon-musk-has-a-big-dream-to-power-tesla-cars-but-reality-may-be-rough-cut- 41598073739715.html.
14 Dale Hall and Nic Lutsey, “Effect of battery manufacturing on electric vehicle life-cycle greenhouse gas emissions,” The International Council on Clean Transportation, February 9, 2018,
https://theicct.org/publications/EV-battery-manufacturing-emissions.
15 Volkswagen, “From the well to the wheel,” April 24, 2019,
https://www.volkswagen-newsroom.com/en/stories/from-the-well-to-the-wheel-4888. Jonathan Eckart, “Batteries can be part of the fight against climate change—if we do these five things,” World Economic Forum, November 28, 2017, https://www.weforum.org/agenda/2017/11/battery-batteries-electric-cars-carbon-sustainable-power-energy/.
16 U.S. Environmental Protection Agency, “Renewable Fuel Standard Program,” accessed September 18, 2020, https://www.epa.gov/renewable-fuel-standard-program/overview-renewable-fuel-standard.
17 David DeGennaro, “Fueling Destruction: The Unintended Consequences of the Renewable Fuel Standard on Land, Water,
and Wildlife,” National Wildlife Federation, 2016,
https://www.nwf.org/~/media/PDFs/Education-Advocacy/Fueling-Destruction_Final.ashx. Joseph Fargione, Jason Hill, David Tilman2, Stephen Polasky, and Peter Hawthorne, “Land Clearing And the Biofuel Carbon Debt,” Science, Vol. 319, Issue 5867 (February 29, 2008), pp. 1235-1238, https://science.sciencemag.org/content/319/5867/1235?ijkey=0a1dec159e875e7ac881b5fa1dc764e2fec50abb&ke ytype2=tf_ipsecsha.
18 Ned Mamula, “Federal Land Withdrawals: Endangering the Nation,” Green Watch, Capital Research Center, January 2020,
https://capitalresearch.org/article/federal-land-withdrawals-part-1/.
19 Ned Mamula, “America’s Rare Earth Ultimatum: Rare Earths in High Demand,” Green Watch, Capital Research Center, May 28, 2019, https://capitalresearch.org/article/americas-rare-earth-ultimatum-part-1/.
20 Trout Unlimited, National Wildlife Federation, Backcountry Hunters and Anglers, Critical Minerals Report: A Conservation
Perspective, August 2020, https://www.tu.org/wp-content/uploads/2020/08/Critical-Minerals_Interactive.pdf.
21 U.S. Army Corps of Engineers, Pebble Project Final Environmental Impact Statement, July 2020, https://pebbleprojecteis.com/documents/finaleis.
22 Taryn Kiekow Heimer, “Pebble Mine: Conservation Groups Sue Trump Administration,” Expert Blog, Natural Resources
Defense Council, October 9, 2019,
https://www.nrdc.org/experts/taryn-kiekow-heimer/pebble-mine-conservation-groups-sue-trump-administration.
23 Council on Environmental Quality, “Update to the Regulations Implementing the Procedural Provisions of the National Environmental Policy Act,” Federal Register, Vol. 85, No. 137 (July 16, 2020), pp. 43304-43376, https://www.govinfo.gov/content/pkg/FR-2020-07-16/pdf/2020-15179.pdf.
24 Council on Environmental Quality, “Environmental Impact Statement Timelines,” December 14, 2018,
https://www.whitehouse.gov/wp-content/uploads/2017/11/CEQ-EIS-Timelines-Report.pdf.
25 National Mining Association, “Permitting, Economic Value and Mining in the United States,” June 19, 2015, http://mineralsmakelife.org/assets/images/content/resources/SNL_Permitting_Delay_Report-Online.pdf.
26 Veronica Stracqualursi, “Environmental Groups Sue over Trump’s Highly Touted Changes to Landmark Regulations,” CNN,
August 6, 2020, https://www.cnn.com/2020/08/06/politics/lawsuit-national-environmental-policy-act/index.html.
27 U.S. Energy Information Administration, “Frequently Asked Questions,” https://www.eia.gov/tools/faqs/faq.php?id=23&t=10#:~:text=In%202019%2C%20about%20142.17%20billion,9.27%20 million%20barrels)%20per%20day.
28 American Petroleum Institute, “Service Station FAQs,” accessed September 18, 2020,
https://www.api.org/oil-and-natural-gas/consumer-information/consumer-resources/service-station-faqs#:~:text=How%20 many%20service%20stations%20are,stations%20across%20the%20United%20States.
29 U.S. Energy Information Administration, “EIA Annual Energy Outlook 2018,” February 6, 2018,
https://www.eia.gov/outlooks/aeo/pdf/AEO2018.pdf. Lesser et al.
30 Allison Weis, Paulina Jaramillo, and Jeremy Michalek, “Consequential life cycle air emissions externalities for plug-in electric vehicles in the PJM interconnection,” Environmental Research Letters, February 9, 2016, https://iopscience.iop.org/article/10.1088/1748-9326/11/2/024009/pdf. Stephen P. Holland, Erin T. Mansur, Nicholas Z. Muller, and Andrew J. Yates, “Environmental Benefits From Driving Electric Vehicles,” National Bureau of Economic Research, June 2015, https://www.nber.org/papers/w21291.pdf.
31 Dan Shreve, “Deep decarbonisation: the multi-trillion dollar question,” Wood Mackenzie, June 27, 2019,
https://www.woodmac.com/news/feature/deep-decarbonisation-the-multi-trillion-dollar-question/.
32 U.S. Energy Information Administration, “Energy Outlook 2020,” January 29, 2020, p. 62, https://www.eia.gov/outlooks/aeo/pdf/aeo2020.pdf.
33 Lesser et al.
34 Ibid.
35 Marco Miotti, Geoffrey J. Supran, Ella J. Kim, and Jessika E. Trancik, “Personal Vehicles Evaluated Against Climate Change Mitigation Targets,” Environmental Science & Technology, September 27, 2016, https://pubs.acs.org/doi/pdf/10.1021/acs.est.6b00177.
36 Shreve, p. 6.
37 Will Wade and Brian Eckhouse, “California Doomed to Frequent Blackout Risk by Battery Shortage,” Bloomberg, August 19, 2020,
https://www.bloomberg.com/news/articles/2020-08-19/california-doomed-to-frequent-blackout-risk-by-battery-shortage. Jeff St. John, “California’s Shift from Natural Gas to Solar Is Playing a Role in Rolling Blackouts,” Green Tech Media, August 17, 2020,
https://www.greentechmedia.com/articles/read/how-californias-shift-from-natural-gas-to-solar-is-playing-a-role-in-rolling- blackouts.
38 Shreve, p. 6.
39 U.S. Environmental Protection Agency, “Control of Air Pollution From Motor Vehicles: Tier 3 Motor Vehicle Emission and Fuel Standards,” April 28, 2014,
https://www.epa.gov/regulations-emissions-vehicles-and-engines/final-rule-amendments-related-tier-3-motor-vehicle. U.S. Environmental Protection Agency and National Highway Traffic Safety Administration, “The Safer Affordable Fuel-Efficient (SAFE) Vehicles Rule for Model Years 2021-2026 Passenger Cars and Light Trucks, April 30, 2020, https://www.govinfo.gov/content/pkg/FR-2020-04-30/pdf/2020-06967.pdf.
40 Gautam Kalghotgi, “The Battery Car Delusion,” The Global Warming Policy Foundation, 2020, https://www.thegwpf.org/content/uploads/2020/07/The-Battery-Car-Delusion.pdf.
41 Ibid.
42 Statement of James J. Greenberger, NAATBATT International, before the Senate Committee on Environment and Public Works, July 17, 2019,
https://www.epw.senate.gov/public/cache/files/e/5/e5530917-434d-451c-8a6b-c5cdfad1b5ec/EED12407A6BF7DE6C86 A4B39C25CF6A4.greenberger-testimony-07.17.2019.pdf. 43 Cassidy Shell, “EV Batteries: Creating A Circular Economy,” Cleantech Group, May 2020, https://www.cleantech.com/ev-batteries-creating-a-circular-economy/. 44 James Delong, “Wasting Away: Managing Municipal Solid Waste,” Competitive Enterprise Institute, 1994. 45 Mitch Jacoby, “It’s time to get serious about recycling lithium-ion batteries,” Chemical & Engineering News, July 14, 2019, https://cen.acs.org/materials/energy-storage/time-serious-recycling-lithium/97/i28. 46 Gavin Harper et al., “Recycling lithium-ion batteries from electric vehicles,” Nature, Vol. 575 (November 7, 2019), https://www.nature.com/articles/s41586-019-1682-5.pdf. 47 Alexandre Beaudet, François Larouche, Kamyab Amouzegar, Patrick Bouchard, and Karim Zaghib, “Key Challenges and Opportunities for Recycling Electric Vehicle Battery Materials,” Sustainability, July 20, 2020, https://www.researchgate.net/publication/343087669_Key_Challenges_and_Opportunities_for_Recycling_Electric_Vehicle Battery_Materials.
48 Dale Hall and Nic Lutsey, p. 8. Rebecca Ciez, et al., “Examining different recycling processes for lithium-ion batteries,”
Nature Sustainability, Vol. 2, Issue 2 (February 11, 2019), pp. 148-156, https://www.nature.com/articles/s41893-019-0222-5.
49 Mills, p. 12.